Overview
Gate-defined quantum dots store qubits in spin states of individual electrons or holes confined by potential landscapes that are shaped by voltages applied to electrodes. Semiconductor quantum dot platform has many favorable attributes for realizing scalable and individually addressable integrated quantum system owing to (1) spin’s long coherence time, (2) potential scalability including well-established fabrication technology, and (3) high degree of Hamiltonian parameter tunability. Depending on the degree of spin-charge mixing, elementary quantum operations of Loss-DiVincenzo (single electron, purely magnetic control), singlet-triplet (ST0, two-electron, electric control under magnetic field gradient), and various three electron spin qubits (all-electric control) have been demonstrated so far.
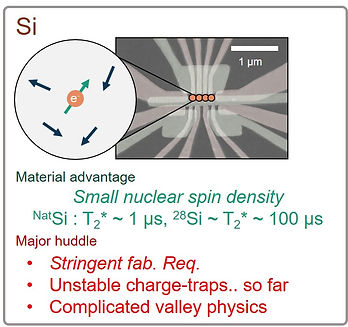

While we are interested in all of the qubit types above, DKim lab at SNU currently focuses on optimizing two-electron singlet-triplet qubits in both silicon and GaAs material system (see above for material choice). Despite added device complexity compared to single-electron spin qubits, that is, one qubit per two coupled quantum dots instead of one quantum dot, this type has many merits including fast electrical spin controls in the presence of magnetic field gradients, large extended sweet spot insensitive to external charge fluctuations, and favorable low operating frequency below 1 GHz enabling relatively easy integration of crowded control lines for multi-qubit operations. Below we describe details of selected sub-topics.
Robust quantum control

Project description: Fidelity of a quantum logic gate is inevitably affected by various decoherence channels due to interaction with environments. Using spin qubits, we develop novel quantum dot tuning methodology, optimal pulse sequences, gate stack geometry, and verification protocols toward building improved physical qubits in semiconductors. The project also includes combining modern machine learning methods to aid in designing control sequences and compressive schemes for quantum state and process tomography.
High-fidelity quantum measurements

Project description: High-fidelity single-shot measurement is an essential requirement for realizing, for example, quantum non-demolition measurement and quantum error correction. In semiconductor quantum dots, the spin-to-charge conversion is the primary method to readout individual spins. We optimize various known methods like Pauli-spin-blockade, energy selective tunneling, and tunneling rate dependence, and develop combined ways to enable fast and high-fidelity projective measurements in semiconductors. The project also includes developing novel cryogenic electronics like rf-reflectometry, ultra-low power CMOS amplifiers for enhancing the sensitivity of the charge sensor.
Measurement-based feedback

Project description: In most material platform for semiconductor quantum dots, qubit frequency fluctuating in time often dominates achievable coherence time. For example, nuclear spin fluctuation, known as Overhauser fluctuation, in GaAs, slow stochastic frequency jumps due to charge fluctuators in MOS-silicon, or even drifts in control electronics, to name a few. We develop primarily with the aid of FPGA (field-programmable-gate-array) programming, to enable real-time Hamiltonian parameter (like qubit frequency) estimation and active measurement-based feedback to suppress relatively low-frequency noise sources. The project also includes further adapting these fast feedback schemes to use in parity measurement based quantum error correction.