top of page
The three dimensional strong topological insulator (STI) is a new phase of electronic matter which is distinct from ordinary insulators in that it supports on its surface a spin-dependent conducting two-dimensional surface state, whose existence is guaranteed by the topology of the band structure of the insulator. The representative STI material is Bi2Se3, which has a bulk band-gap of 300 meV, much greater than room temperature, and a single topological Dirac surface state. It has been first theoretically shown that the topologically protected metallic surface states can provide rich system to observe quantum mechanical quasi particles or collective excitations that are fundamentally different from ordinary metals or insulators, and the proposer’s past works mainly focused on the electrical and thermal transport measurement on the surface of STIs. Among these exotic properties of surface states of STI, the surface electron’s ‘spin-momentum helicity’ renders spin and momentum perfectly locked together. Since electron’s average momentum can be controlled by electric potential, spin-momentum helicity can potentially lead to direct electric control of surface spins, which is essential development needed in the field of spintronics. We propose and perform a research to experimentally confirm spin-polarization of surface electrons of 3D topological insulators. Special emphasize is put on the measurement of spin-polarization by means of electronic transport relevant to the most of device applications.
Due to its spintronic device potential at room temperature, there has been great interests in the measurement of spin-polarization of electrons on the surface of STIs, and representative results are obtained by Spin-Angle Resolved Photo Emission Spectroscopy (spin-ARPES) technique. These studies confirmed that indeed electrons have high-degree of spin-polarization in the surface of STIs. There has been several attempts to argue the evidence of surface spin polarization by measuring magneto resistance (MR) effect between ferromagnetic (FM) contacts formed on the surface of STI. While these studies demonstrated indirect evidence and possible device application potential at room temperature, these measurement could not reveal clear and direct signature of surface spin transport for several reasons. First of all, since spin and momentum are perfectly coupled, non-local measurement of a spin current in the absence of a charge current is precluded on the surface of STIs. Even more importantly, spin precession induced by a weak perpendicular magnetic field is also eliminated by momentum scattering in the diffusive regime. This is particularly problematic since evidence of spin precession and dephasing are used to unambiguously identify spin transport in both inorganic semiconductors and metals. Moreover, in-plane magnetic field geometry or making use of tunneling junctions are unlikely to solve the problem because of planar Hall effect or spurious signal due to strong spin-orbit coupling of STIs that are difficult to distinguish from electronic spin injection signals from FM contacts to STI surfaces.
We design a class of transport experiments to confirm the spin-momentum coupling in STI surface states which circumvents aforementioned problems. Since conventional smoking gun signature of spin transport is forbidden in STI surfaces, the key approach involves injection of spin polarized electrons from the topological insulator to non-magnetic spin transport channel, such as graphene, and confirm spin polarization by spin precession measurement of the transferred electrons. We plan to develop and test various forms of topological insulator/non-magnetic material hetero-structures which can enable electrical measurement of current induced spin polarization of STIs. A most probable scheme is to use mono/few layer graphene as a spin transport channel.
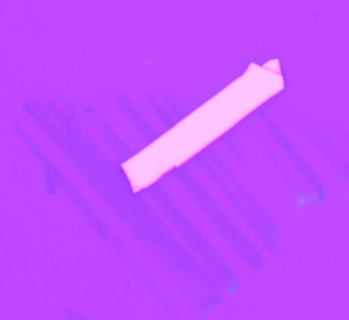
Figure 1. An example of stacked graphene/Bi2Se3 heterostructure
The use of graphene has several advantages compared to other low dimensional materials : (1) absence of Schottky barrier in STI/graphene junction since graphene is known to be a gapless semimetal, (2) no conductivity mismatch problem which enables efficient spin injection, and (3) simple fabrication method as various techniques for making graphene hetero-structures are available. Moreover, graphene, similar to Si, has low spin-orbit coupling and has been shown to have spin diffusion length order of micro meters at room temperature. Therefore the fabrication of FM electrode on the graphene does not require nanometer scale positioning resolution. An alternative way to probe spin polarized current is to investigate photocurrent in STI induced by circularly polarized light. Both theoretical and experimental studies suggest that angular momentum of the circularly polarized light can preferentially couple to one spin branch of topological surface electrons, and STI can exhibit novel photo-galvanic effect, such as helicity dependent photo current (HDPC) that is normally absent in inversion symmetric crystals. Topological insulator is also expected to act as efficient spin detector through surface spin momentum locking, thus completely non-ferromagnetic spin injection and detection is also plausible. Our group explore these device configurations and actively searhc for spin transport signal in TIs.

Figure 2. A TI/Gr device on hexagonal Boron Nitride - device by Dr. Son
bottom of page